· Physics · 5 min read
Quantum Chromodynamics: Unlocking the Secrets of Strong Force
Quantum chromodynamics unravels the secrets of the strong force, binding particles with intriguing interactions. Dive deep into the forces shaping atomic nuclei.
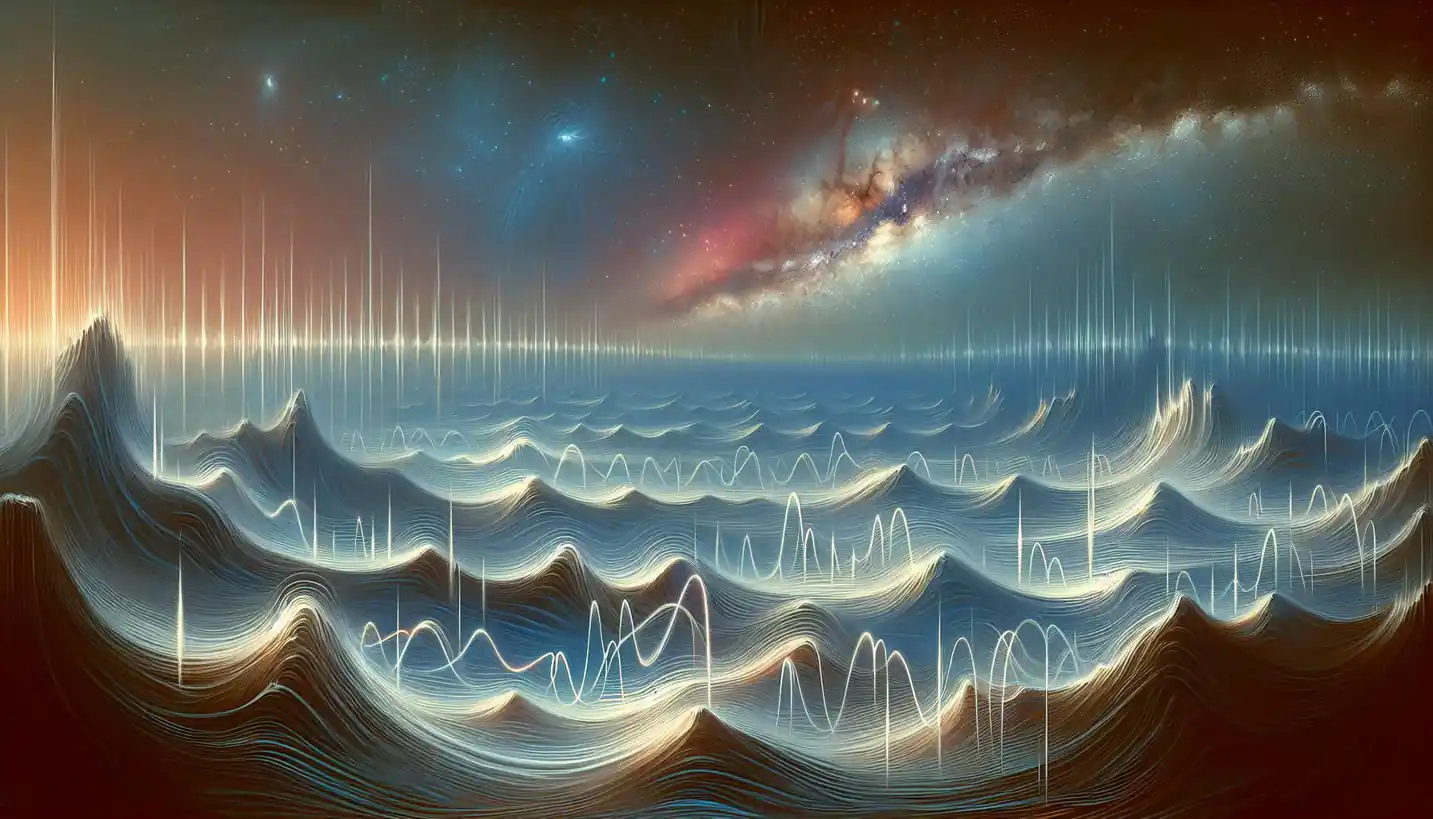
Once upon a time in the world of tiny particles, scientists were puzzling over what held the atomic nucleus together. They knew about the strong nuclear force, but couldn’t quite pin down how it worked. Eventually, they unearthed a theory called Quantum Chromodynamics (QCD), which offered a compelling explanation for this enigmatic force.
Imagine you’re looking at a bustling city intersection. Cars and pedestrians mingle and move around, all following traffic rules to keep chaos at bay. In the microscopic world of physics, particles like protons and neutrons are in constant motion too, and QCD is the rulebook that dictates how they interact.
What is Quantum Chromodynamics?
At the heart of QCD are quarks and gluons. Quarks are like the colorful building blocks of matter, coming in six types or “flavors”: up, down, charm, strange, top, and bottom. These flavors are whimsically named but play a crucial role in forming protons and neutrons, the particles that make up the nucleus of an atom.
Gluons, on the other hand, act like the “glue” that binds quarks together. They’re the messengers of the strong force, keeping quarks in check and making sure they don’t fly apart. If we think of quarks as dancers on a stage, gluons are the invisible strings that guide their steps.
The Colors of Quarks
In QCD, quarks possess a unique property called “color charge,” but don’t worry, it has nothing to do with actual colors. It’s just a clever analogy to describe how quarks interact with each other. There are three “colors”: red, green, and blue, and these combine in various ways to form a “white” or neutral state, much like how the primary colors of light combine.
This color charge is fundamental to how quarks bond. For example, in a proton, you’ll find three quarks, each of a different color, bound together by gluons. Nature seems to have a rule: you can’t find a lone quark by itself; it’s always paired up with others to balance its color charge.
How Gluons Tie Everything Together
Gluons are fascinating because they aren’t just ferrying messages between quarks; they also carry a color charge themselves. This means gluons can interact with one another, making QCD intensely complex. It’s like a dance where not just the dancers but also the dance floor itself is shifting and evolving.
One of the most intriguing aspects of QCD is “asymptotic freedom.” This concept might sound like something out of science fiction, but it means that the closer quarks get to each other, the weaker their interaction becomes. Conversely, as they move apart, the force between them strengthens. Imagine two magnets that repel each other more strongly as they’re pulled away – it’s counterintuitive but absolutely vital for the stability of matter.
The Mysteries and Challenges of QCD
Though it’s a cornerstone of the Standard Model of particle physics, QCD isn’t easy to visualize or test directly. Because the strong force is so powerful, experiments must be incredibly precise and conditions controlled just right to observe its effects. This is why massive particle colliders, like the Large Hadron Collider, play such a crucial role. They recreate the conditions needed to study quarks and gluons in action.
The unpredictable nature of quarks and gluons sometimes leads to colorful phenomena called “jets,” where particles are emitted in tight streams. This is part of what makes studying QCD both fascinating and challenging. Researchers use a method called “lattice QCD,” where they simulate quark interactions on a grid-like structure, much like pixels on a screen, to try to predict these behaviors.
Why QCD Matters
You might wonder why understanding QCD is important. First, it helps us comprehend the very foundation of matter and the forces that hold it together. It gives insight into the early universe, moments after the Big Bang, where conditions were so hot and dense that understanding the behavior of quarks and gluons becomes crucial.
Moreover, QCD is essential in practical applications, from developing new technologies to medical advancements like cancer treatment. Techniques that emerged from particle physics, such as PET scans, rely on insights gained from studying the fundamental forces and particles.
The Future of QCD Research
As our tools and techniques improve, the mysteries of QCD become more accessible. Future research may unlock secrets about the nature of dark matter or reveal new particles and forces. With each discovery, we not only expand our understanding of the universe but also open doors to new technologies and innovations.
QCD represents a beautiful fusion of complexity and simplicity. It reminds us that even in the unseen corners of the universe, rules and patterns emerge, guiding the dance of reality. As we continue to probe deeper into the quantum realm, we take one step closer to deciphering the universe’s ultimate secrets. So next time you look up at the stars, remember the tiny, colorful quarks and gluons, dancing to the tune of quantum chromodynamics, shaping the cosmos as we know it.