· Physics · 5 min read
Damping: The Unsung Hero of Classical Mechanics
Damping, the unsung hero, reduces vibrations and stabilizes systems. Explore its crucial role in classical mechanics and everyday technology.
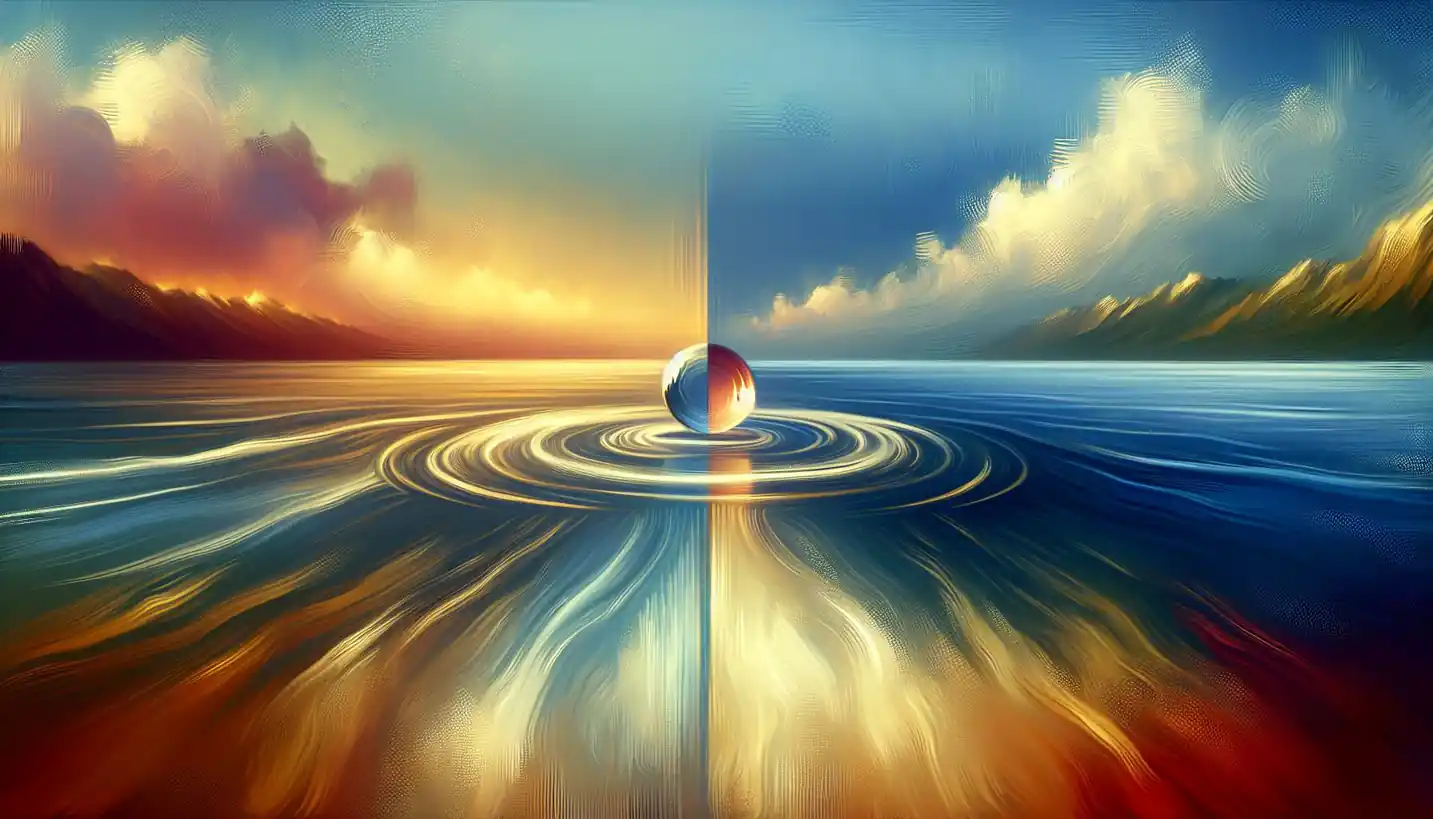
Ever drop something heavy on your foot and notice how it doesn’t bounce back as a rubber ball would? That’s all thanks to a concept called damping. In the world of classical mechanics, damping plays a crucial role in controlling the motion of objects. Let’s delve into this concept and unwrap its significance in our everyday life.
Imagine you’re pushing a child on a swing. Initially, the swing goes high, but if you stop pushing, it gradually slows down and eventually stops. This slowing down isn’t because of the child deciding to quit mid-air; it’s due to damping. Damping refers to the forces that reduce the energy of motion in systems, causing them to slow down over time.
The Role of Damping in Our World
Damping is everywhere—from the squeaky door that slowly shuts to the shocks in your car that absorb bumpy roads. Consider a guitar string that vibrates when plucked. Without damping, the sound would carry on indefinitely. Thanks to damping, it gradually fades, giving us the beautiful decay of a note. It’s not magic—it’s physics at work.
Why Damping Matters
To understand why damping is essential, we first need to look at its role in stability and safety. Damping helps in preventing systems from going haywire. Think of airplanes or bridges; without adequate damping, the vibrations caused by wind or movement could lead to catastrophic failures. Imagine being on a plane where the wings just keep flapping excessively—the thought alone is unsettling!
Different Flavors of Damping
Just like ice cream, damping comes in various types, each with a unique flavor (or function). The main ones are:
Viscous Damping: This is the most common type. Picture moving through honey; the resistance you feel is akin to viscous damping. It acts proportionally to the velocity of the object.
Coulomb or Dry Damping: Here, the frictional forces between surfaces in contact come into play. It doesn’t matter how fast you’re moving; the force remains constant.
Structural Damping: Common in materials that flex, like metals. Think about repeatedly bending a paper clip. It doesn’t revert to its original shape completely because of internal friction.
Radiation Damping: This is more of a player in the vast cosmos. It refers to the energy lost by systems, like electrons, in the form of radiation.
Real-Life Examples of Damping
Let’s put these into perspective with real-life examples. Every time you adjust your radio’s volume using a knob, damping ensures it doesn’t keep spinning indefinitely. Shock absorbers in cars? Viscous damping at its finest, allowing for a smoother ride.
Buildings and bridges use damping to withstand earthquakes. Engineers design structures with specific damping mechanisms to absorb and dissipate seismic energy, reducing the chances of collapse.
The Science Behind Damping
The math behind damping involves a second-order differential equation, which describes how the force of damping is related to the velocity or displacement of the oscillating system. Though it might sound complicated, it boils down to energy reduction and stabilization.
Let’s take the equation of motion for a damped harmonic oscillator:
[ m\ddot{x} + c\dot{x} + kx = 0 ]
- ( m ) is the mass,
- ( c ) is the damping coefficient,
- ( k ) is the spring constant,
- ( x ) is the displacement.
The damping coefficient, ( c ), tells us just how much damping is affecting the system. Larger ( c ) implies more damping.
Overdamping, Underdamping, and Critical Damping
The damping level affects how an object behaves post-disturbance:
- Underdamping: The system oscillates with gradually reducing amplitude. This is common in most systems.
- Overdamping: The system returns to equilibrium slowly without oscillating. Think of a door with a very heavy damper—it closes without bouncing back.
- Critical Damping: The sweet spot. The system returns to rest in the shortest time without oscillating. This is often the goal in engineering systems.
Damping in the Future
As we look towards the future, damping remains a field ripe for innovation. In technology, as electronics get smaller, damping is crucial in managing thermal energy and vibrations.
Imagine the future of space travel. Radiation damping will play a significant role in spacecraft designed to travel across galaxies. Engineers will have to think about how to manage the energy lost through radiation in vast space environments.
The Human Touch in Damping
The concept of damping isn’t just a cold, mechanical one. It’s intertwined with our quest for efficiency and safety. By understanding and utilizing damping, we can create marvels—from silent electric motors to earthquake-resistant skyscrapers.
Open-ended questions like, “How can we enhance damping materials to better mimic natural processes?” keep scientists and engineers up at night. By exploring these avenues, we could unlock new technologies that are safer, quieter, and more efficient.
Conclusion
In essence, damping, an unsung hero in the book of classical mechanics, continues to shape the world around us. It’s that subtle force that doesn’t seek attention but ensures our lives run smoothly. From the ebb of a musical note to the stability of towering structures, damping quietly holds the fabric of our technological environment together.
The next time you appreciate a gentle, unobtrusive motion or a stable structure, give a nod to damping. It’s a reminder of the elegant dance between motion and stillness that defines much of our universe. How can further exploration of this concept lead to advancements in our understanding and technology? The doors are open—what lies beyond is only limited by our imagination.